DMREF Project Develops Advanced Material to Efficiently Store Solar Energy
The team’s fundamental research brings us one step closer to reducing our reliance on fossil fuels.
By Divya Abhat
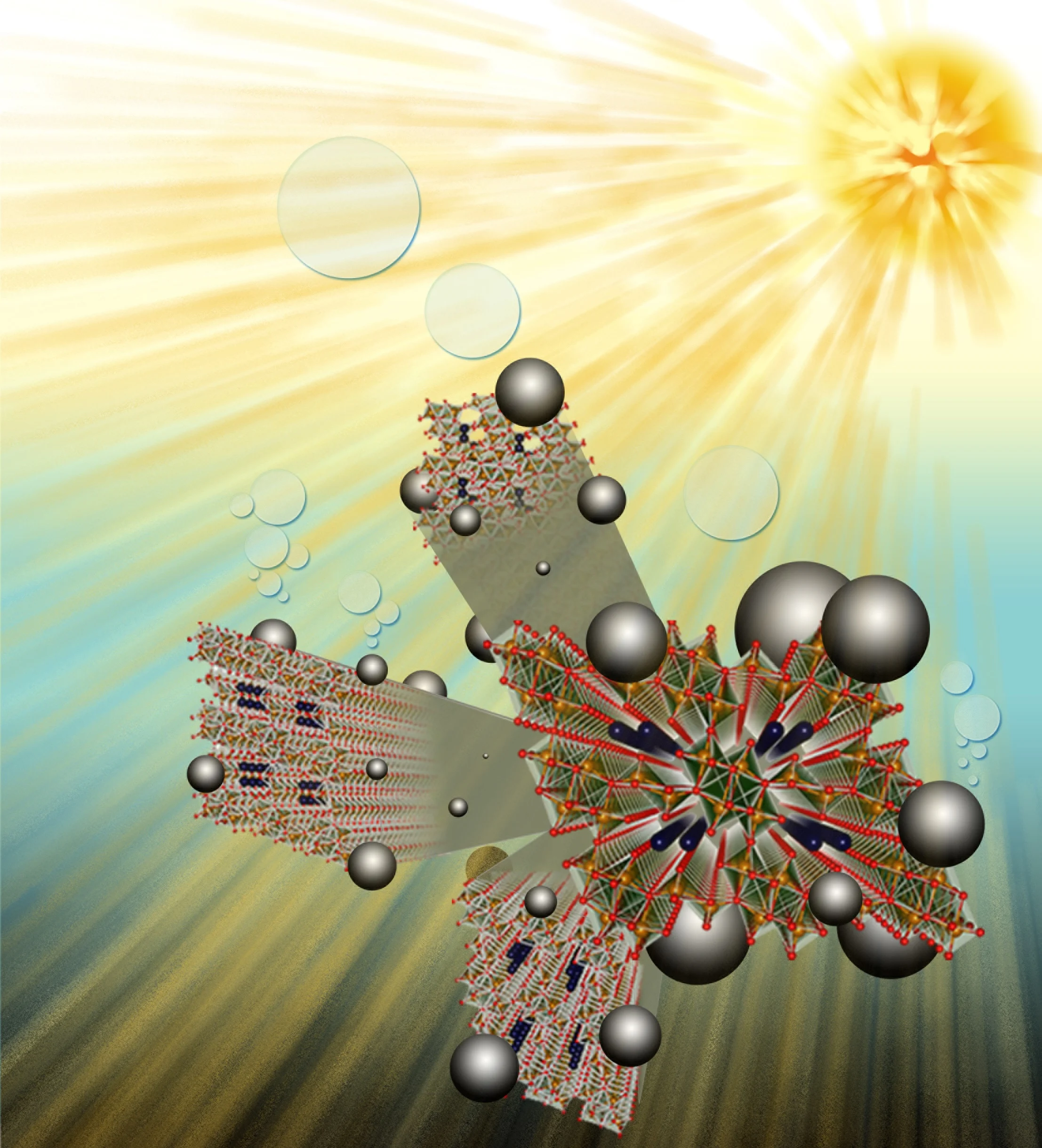
Sunlight is an abundant renewable energy source, but its availability varies with the time of day and weather conditions, which can impede efforts to store this energy for consistent use.
As a solution, a DMREF team, led by David Watson, a chemistry professor at the University at Buffalo, has focused its research on photocatalytic water splitting—a process that involves splitting water into hydrogen and oxygen, which in turn produces “solar-generated fuels.” Hydrogen, when burned, releases energy and produces only water as a byproduct—thus avoiding harmful greenhouse gas emissions typically associated with burning fossil fuels.
It’s all geared towards solar energy conversion as a way to decrease humanity’s reliance on burning fossil fuels,” says Watson. “What we’re doing is completely fundamental research … but it’s absolutely motivated by that goal to have a sustainable alternative—in this case, solar-powered ways to make useful fuels.
To use sunlight to split water, a photocatalyst system must effectively manage a complicated series of steps: First, the system must capture sunlight effectively. Once sunlight is absorbed, it creates pairs of electrons and positively charged holes. These need to be separated quickly to prevent them from recombining, which would waste the absorbed energy. Next, the separated electrons and holes must be moved to specific parts of the catalyst where they can drive chemical reactions. These reactions involve multiple steps and changes in electron arrangements, which split water into hydrogen and oxygen.
It’s unlikely that a single material could manage all of these complex processes alone. Instead, hybrid heterostructures—made from a combination of materials—are used to make the system more effective at using sunlight to split water.
The team has focused on finding the right combination of materials to develop an efficient catalyst system. They’ve used materials abundant in the earth’s crust—an approach that enhances viability for widespread use and reduces dependency on critical material supply chains that can be impacted by geopolitical fluctuations. To efficiently filter the vast array of potential compositions, the team has established a rigorous iterative feedback loop between theoretical and experimental methods, aligning with the core principles of the Materials Genome Initiative (MGI).
This approach to accelerated materials research has paid off: Recently, the team created a new photocatalyst by combining quantum dots with a specific type of semiconductor Sb2VO5—a new and improved combination of materials that is extremely efficient at moving electrical charges, which is crucial for speeding up chemical reactions needed to create fuel from light. They have also developed a way to finely control how charges move and how reactions occur in another set of materials—referred to as Quantum Dot-MoS2 heterostructures—that directly produce hydrogen from water when exposed to light, without needing additional substances to help the reaction along.
Simultaneously, the team has computationally modeled the charge-transfer processes that underpin photocatalysis.
The Sb2VO5 material has yielded our newest interfaces with the most optimal energetics yet,” says Watson. “And then the MoS2 linkage gets us to our best performance on the other end schematically, our most rapid and efficient reduction of H+ (a hydrogen ion or proton) to hydrogen. So, all together these recent advancements make the photocatalysts work better and better.
The Role of Machine Learning and Artificial Intelligence
Another compelling component of this project is its pioneering use of machine learning to design chemical syntheses, allowing researchers to predictively control aspects of material creation, such as the thickness of layers at a very fine scale.
The research combined advanced techniques from powerful synchrotron radiation facilities in the U.S., Canada, and the U.K., along with new X-ray methods developed at Binghamton and Texas A&M University. These approaches helped identify the best composition and structure for the MxV2O5 nanowires to work effectively with the quantum dots. The theoretical calculations and simulations that were performed supported the experimental findings and helped clarify why certain materials worked better than others in practical tests.
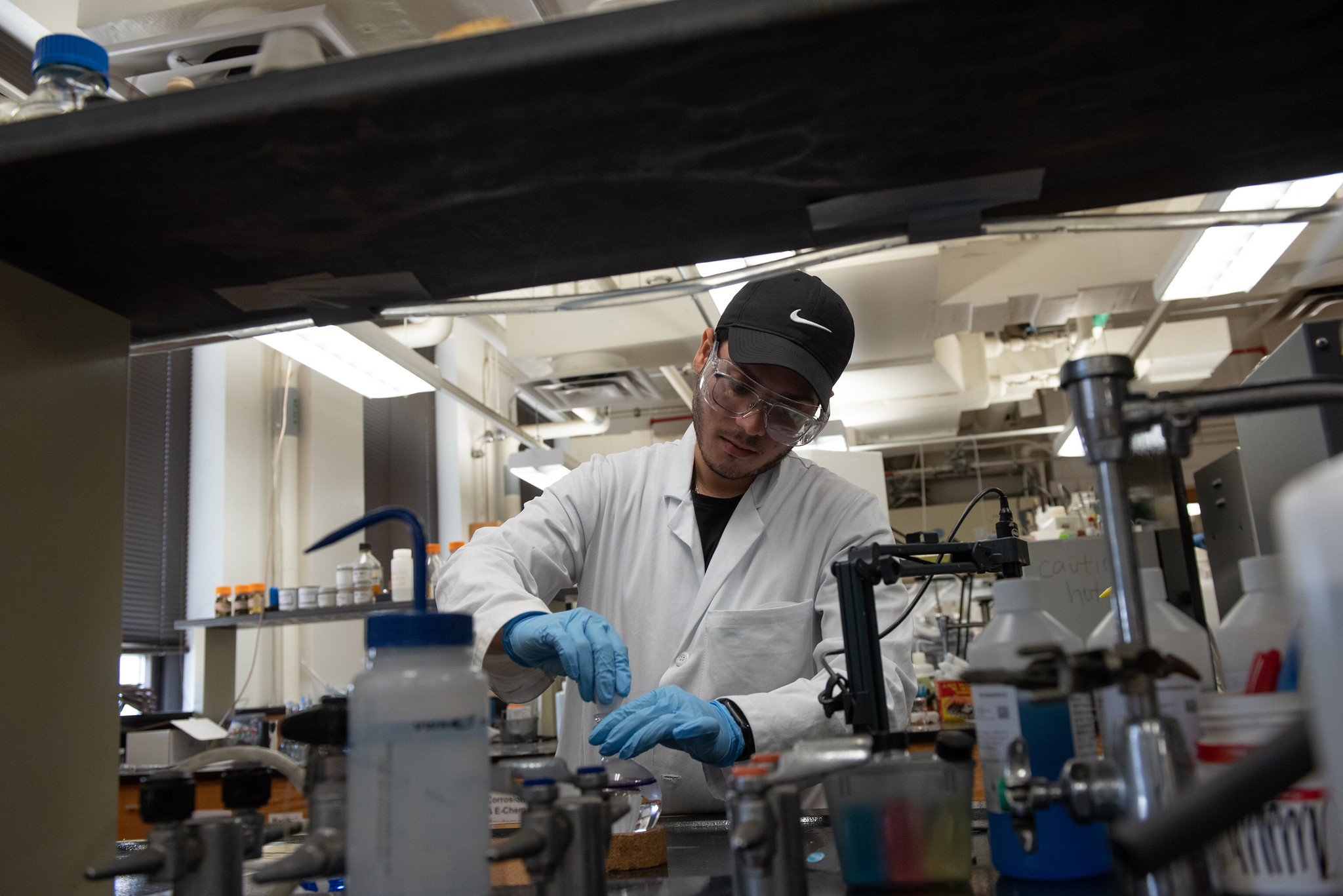
“As an example, we have developed a machine-learning- and deep-learning-aided workflow to track the insertion of lithium ions in V2O5 particles and to map how the material is transformed, monitor stresses generated within particles that could lead to failure, and rapidly identify the best possible shapes and sizes of metal-intercalated vanadium oxide (MxV2O5) particles that can be integrated within electrodes,” says Sarbajit Banerjee, a chemistry professor at Texas A&M and researcher on the team.
Further experiments extended these findings by connecting quantum dots to other nanomaterials, like MoS2, using similar design principles to further enhance performance.
This work has led to significant developments, including the establishment of new X-ray research facilities at Binghamton University, machine-learning workflows to process X-ray maps and electron microscopy images in real-time, and several high-profile publications.
Collaboration and Workforce Development
The DMREF project is co-funded by four NSF Divisions: Materials Research (DMR); Chemistry (CHE); Mathematical Sciences (DMS); and Chemical, Bioengineering, Environmental and Transport Systems (CBET). The team—comprised of five lead researchers along with several graduate and postgraduate students—underscores the power of collaboration. Together, they have published more than 50 papers since the project’s launch in 2016 and have numerous awards and accomplishments under their belt including the prestigious DMREF Creativity Extension—a mechanism by which outstanding teams can be recognized and rewarded while enabling them to carry their research in bold new directions.
“It is really rewarding to see what a multidisciplinary team of materials scientists, chemists, mathematicians, data scientists, and engineers can accomplish when they work together on a complex problem,” says John Schlueter, DMREF Program Director.
The team formed when Peihong Zhang, a physics professor at the University at Buffalo, joined forces with Banerjee and Louis Piper, formerly from Binghamton University and now at the University of Warwick, to synthesize a range of MxV2O5 materials and to predict and measure the unique electronic structure of these new materials.

Watson and Banerjee’s teams combined tiny light-absorbing quantum dots with various MxV2O5 materials—looking closely at how these new combinations reacted when exposed to light. Specifically, they studied the light-induced charge-transfer and photocatalysis reactivity of the resulting heterostructured photocatalysts. In concert, Shengbai Zhang, a physics professor at Rensselaer Polytechnic Institute, and his team have focused on the computational side of this effort.
Training the Next Generation
The team is also committed to mentoring young scientists from underrepresented groups (URMs) and engages K-12 students and teachers in activities that emphasize opportunities made available by big data and solar energy. In fact, since 2016, Watson and Banerjee have mentored 11 URM undergraduate summer research interns from the University of Puerto Rico Cayey, recruited from materials workshops held at the University.
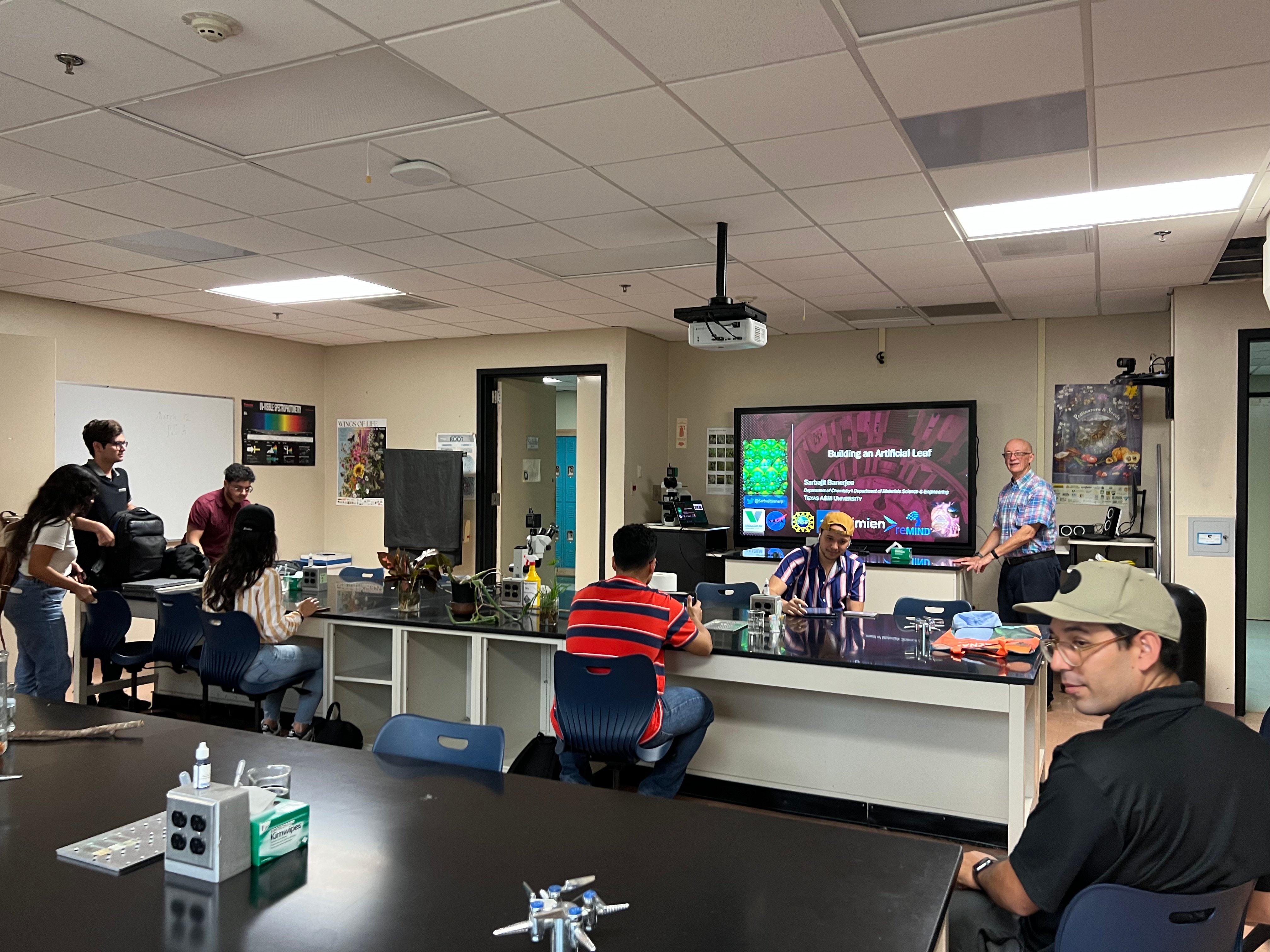
“Our partnership with the University of Puerto Rico has become an exemplar of authentic partnerships between R01 universities and primarily undergraduate institutions and has allowed us to focus on meaningful student outcomes,” says Banerjee.
Several UPRC alumni have earned PhD degrees with the team and have achieved excellent post-PhD outcomes. For instance, Luis De Jesús, a PhD student at Texas A&M who worked with Banerjee recently joined the University at Buffalo’s Department of Chemistry as a tenure-track assistant professor. Similarly, Natalia Rivera-González, who completed her PhD with Watson at the University at Buffalo and did her postdoctoral research at Texas A&M with Banerjee, is now a research scientist at Solvay, a Belgian multinational chemical company. Karoline García-Pedraza, James Vasquez, and Carlos Larriuz, former DMREF summer interns, are currently pursuing PhDs with Watson and Banerjee.
This collaboration project has introduced me to a research interest I did not know I had in me,” says García-Pedraza. “The development of photocatalytic architectures has become a fascinating field for me, creating a curiosity and motivating me to continue my contribution to their development. My research findings have also allowed me to present at several scientific meetings, such as the American Chemical Society, Materials Research Society, and Northeastern Regional Meeting.
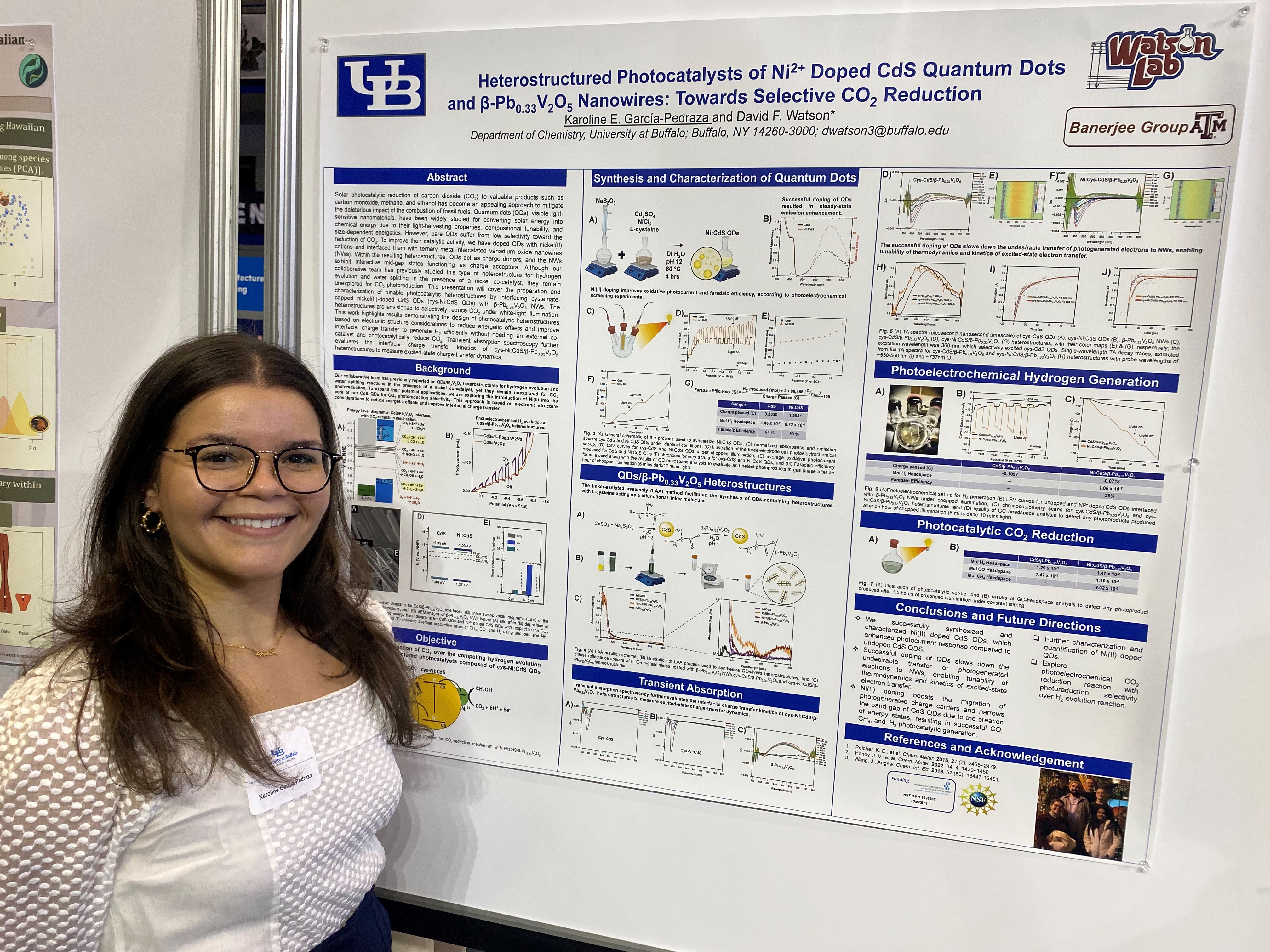
In addition, several graduate research students from the DMREF team have completed Texas A&M’s two-week summer school on Computational Materials Science across Scales, which includes several modules on data science and is preceded by a weekend bootcamp in Python coding at the University.
Next Steps
Beyond foundational research, the team also focuses on practical applications. The researchers are exploring the use of their catalysts for broader chemical processes and have developed special coatings for handling viscous liquids and separating complex mixtures of fluids. In addition, some members of the team have participated in NSF’s Innovation Corps or I-Corps entrepreneurial training program to explore the market potential of their technologies. They are now moving toward commercializing some of these innovations through a recently launched startup located in Katy, Texas.
These developments tie into the broader goals of the project by demonstrating practical applications and integration of innovative technologies to advance solar energy conversion and catalysis, while also training and involving a diverse group of researchers in high-impact scientific work.
This team has really ‘covered all the bases’ with respect to working under the philosophy of the Materials Genome Initiative,” says Schlueter. “These ambitious and forward-looking PIs exemplify what a DMREF team should aspire to achieve.